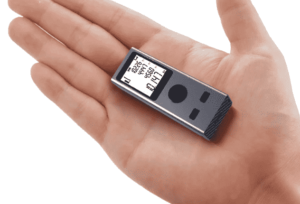
I recently received this handheld distance measurement device. Like a laser pointer, it shoots out a narrow beam of red light, making a small red dot on a surface that might be 100 feet away. It then uses a built-in telescope to view the red dot of light, and works out the distance to that surface. The device arrived today, and I compared its measurement with that of a long fiberglass measuring tape. The two approaches yielded answers that differed by only a tenth of a percent. How does it work exactly?
Star Trek fans might be able to guess where I am going with this. There were episodes of Star Trek where an enemy had somehow found a way to bypass the Enterprise’s shields, at which point the captain on the bridge of the Enterprise would shout out “modulate the shield harmonics!” In the world of Star Trek, this modulation apparently restored the protection of the shields. And indeed this kind of laser distance measurement uses a modulation of a signal to accomplish its remarkable result.
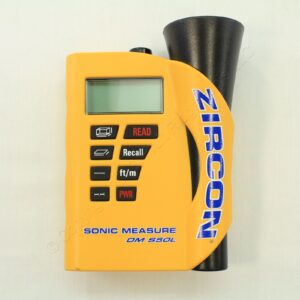
By way of background, the first “electronic measuring tape” device that I ever owned (seen at right) came to me maybe 30 years ago, and it worked by emitting an audible click and listening for the reflected sound of that click. I never went to the trouble to check its accuracy, but it claimed to be accurate within a half of one percent. A second operational mode permitted the user to measure an area of a rectangle by doing two consecutive length measurements (in other words it would multiply the two numbers). A third operational mode permitted the user to measure a volume of a rectangular room by doing three consecutive length measurements (in other words it would multiply the three numbers). Yet another operational mode permitted the user to measure two lengths along a straight path and add the two numbers together to work out the overall length.
The alert reader realizes that the sonic distance measurement device used the speed of sound in its calculations. This means that the interval between emitting the click and receiving the echo was in the nature of some milliseconds. Light travels a lot faster than sound, of course, at about a nanosecond per foot. If the distance being optically measured is, say, 30 feet away, then the “echo” of the emitted photon would be delayed by around 60 nanoseconds. Can it be that the small device shown at the beginning of the article is simply measuring the round-trip time for an emitted blink of light? The answer is “no”. It is simply not practical to try to measure a few tens of nanoseconds to an accuracy of a tenth of a percent.
So how does the optical range finder work? The answer is that the small handheld device pictured above imposes an amplitude modulation upon the emitted laser light. The device detects a phase difference between the emitted and received light signals, and works out the distance by doing some math on the measured phase difference.
The sonic measuring device from 30 years ago claimed to be able to measure things as much as 30 feet away. Of course this worked only if the target was a fairly hard surface. If it were a sound-absorptive surface (covered with drapes or carpet for example) then the device would not have a reflected chirp to listen to.
The laser rangefinder pictured above claims to be able to measure things as much as 100 feet (30 meters) away. The test that I did (the test that turned out to match a physical tape measure to within a tenth of a percent) was to a target that was a matte-finish painted metal railing that was nearly black in color. The target was about ten meters (about 30 feet) away, in a brightly lit room.
After a moment’s reflection (sorry) I realized that I do not really know whether the tenth-of-a-percent difference between the two measured distances (using the fiberglass tape measure and using the optical tape measure) is an error in the optically measured distance. Maybe some of the error comes from the fiberglass tape measure, for all I know. Maybe the optical device is more accurate than the physical tape measure.
The test that I described here, with the red dot of light landing on a dark matte-finish metal railing, was about ten meters (about thirty feet). The maker of the optical device claims it can measure to objects as much as 30 meters (about 100 feet). I have not yet tried this device outdoors during the day. My guess is that in bright sunlight, it probably cannot measure distances that are quite that far away.
The sonic measurement needed to be from a fairly uniform surface like a wall. If you had a goal of measuring a distance to a small object that happened to have a big wall behind it, you would fail at your goal because the echo from the big wall would be louder than the echo from the small object. In contrast, with the optical device, you could point the laser beam toward the small object, and you could measure the distance to the red dot at a moment when the red dot is striking the small object. The presence of a big wall behind the object of interest is not a problem if you are using the optical device instead of the sonic device.
The speed of sound in air is influenced by the humidity of the air and the temperature of the air. This introduces some error in a sonic distance measurement. In contrast, accuracy of the optical device is not thrown off by variations in humidity or ambient temperature.
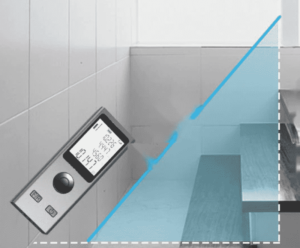
Now let’s suppose your target is at a horizontal distance from your location, but let’s suppose further that you do not have a direct line of sight to the target. I was astonished to find that one of the operational modes for this device, as illustrated at right, is that you can ask the device to measure the hypotenuse of a triangle, and then you can ask it to do a “cotangent” trigonometry calculation to work out the adjacent (horizontal) length of the triangle. Yes, from this we realize that the device must contain a two-axis microelectronic mechanical tilt sensor, and can draw upon a measured angle from the horizontal at the time of the optical distance measurement. (My guess is that the device does not actually evaluate the trig function but instead does a simple lookup in a stored table of trig values.)
When you do a hypotenuse-based distance measurement like this, the device displays the tilt angle on its display. It took me a moment to realize that this means the device can also be used as a digital protractor (you simply ignore the distance information on the display).
The display is, of course, backlit. The device displays the three most recent distance measurement values, which gives you an opportunity to compare them and to write down the number that you want to keep.
Of course the designer of this optical tape measure device also provides operational modes like those of the sonic tape measure device. You can measure the width and length of a room to get the floor area of the room (multiplying two numbers). You can measure three lengths to get the volume of the room (multiplying three numbers). You can measure two lengths along a line to get a total length (adding two numbers). You can measure “out and part way back” to get a net length (subtracting one number from another).
This device weighs only 24 grams (less than an ounce). It is 55 mm (less than 2¼ inches) long and 22 mm (less than an inch) wide. It is 13 mm (about half an inch) thick. It is powered by a (non-replaceable) built-in rechargeable battery. You charge it using an ordinary USB cable.
Oh and of course you get to specify whether the distance reported by the device is counting from the “bottom” or foot of the device on the one hand, or is counting from the “top” or the head of the device on the other hand. In other words you can ask it to subtract its 55-mm length from the measured distance.
It turns out that this device only costs $22, which includes shipping.
I am thinking that in some future blog article I may reveal where I purchased this device. Or maybe some alert reader can figure out where I purchased it.
Bosch/FBLFOBELI sells one for for $15.99 through Amazon and it is advertised to measure up to 165 feet. Made in China, of course.
https://www.amazon.com/dp/B09QBYJ9GK/
What you get for $15.99 is a carrying case for the Bosch device. The Bosch device (Amazon link) costs $120. And the Bosch device is physically bigger than the device in the blog article, being 4.2 inches by 1.8 by 0.9. The volume of the Bosch device is seven times greater (6.8 cubic inches) than the volume of the device in the blog article (less than one cubic inch).
Looks like ETEKJOY — https://www.amazon.com//dp/B0983FH1DF
Pretty reasonable price there!
Yes you are right. This is the exact same product. My source cost $22 to your $29, but yes, I am sure it is the same product.
It is interesting that this device also does not have a speed indicting mode for a moving object. I would think that it wouldn’t be too hard to have its firmware make two measurements a fixed time apart and calculate a speed.
I suspect that part of what is going on is that to do the distance measurement, the device is required to do a quite subtle phase-difference measurement between the outbound photon stream and the bounced-back photon stream, and this probably gets accomplished only if the target is motionless, or nearly so, relative to the device. If one has arbitrarily high processing bandwidth in the device, maybe the phase-difference measurement and associated calculations can be done in real time even for moving targets. But to keep the form factor small and to keep everything within a modest battery budget, and to keep the price down, the designers probably dialed down the processor clock to the lowest possible clock speed, and made the data-collection electronics as slow as they could be consistent with the other design goals. And what one finishes with is a device that works well only if the target is motionless, or nearly so, relative to the device.
Layered on top of this is that for the phase analysis to get accomplished, the red dot is required to be pointed at the target, and is required to have landed on a place on the target that is returning enough photon scatter in the needed direction (on a return path back toward the device). With a stationary target these requirements are within grasp for the alert human user, but it does require some amount of close attention and fairly steady aiming activity. For a moving target, however, these requirements might well be something that even an alert human user might not be able to accomplish reliably.